Laser-driven ion acceleration has recently proven capable of generating mono-energetic ions with characteristics suitable for a variety of applications. Important examples are proton therapy for treatment of deep tumors in cancer patients, and the fast ignition scheme of inertial confinement fusion. In both cases the advantage of using ions, often protons, is their ability to deposit a their kinetic energy in a small target volume, known as the Bragg peak. Laser-plasma interactions can also support accelerating fields orders of magnitude higher than conventional accelerators, and produce beams of much higher intensity.
Acceleration of ions is accomplished with an ultraintense laser incident on an ultrathin foil, which instantly is instantly ionized into an overdense plasma. In the radiation pressure acceleration (RPA) regime, electrons in the foil are pushed via the laser ponderomotive force, and set up the electrostatic acceleration field, which accelerates the ions. Circularly polarized lasers prevent electron heating, as the ponderomotive force is constant in time.
The key challenge is reducing the spread in ion energy. A primary factor in energy spectrum degradation is the stability of the ions. In the ions’ rest frame they are resting on the laser front, and therefore are subject to the classic Rayleigh-Taylor Instability seen when a heavy fluid is positioned above a lighter fluid.
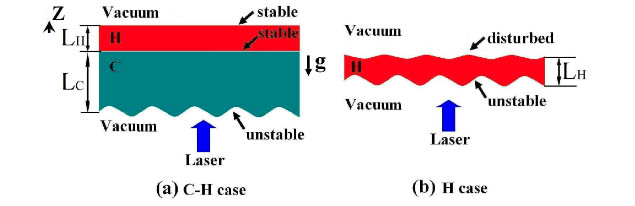
Our work has two thrusts. The first thrust is to develop a self-consistent kinetic theory of a laser-accelerated plasma target with distributed electron/ion. We identified and analytically described several distinct target regions (nonneutral ion tail, non-neutral electron sheath, and neutral plasma bulk) in the target uniformly accelerated by the self-consistent electric field generated by hot electrons trapped in combined ponderomotive and electrostatic potentials moving.
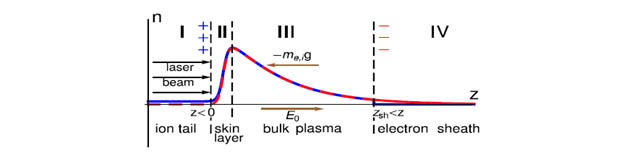
Our next step is generalized a theory for composite multispecies target.
The second thrust is to develop a model of Rayleigh-Taylor Instability for accelerated plasma target. The simplest fluid description of the evolution of small perturbations leads to classical result for the growth rate of the instability $\gamma \propto k^{1/2}$. However PIC simulations of the evolution of seeded target perturbations shows that their growth rate can be significantly less than predicted by theory.
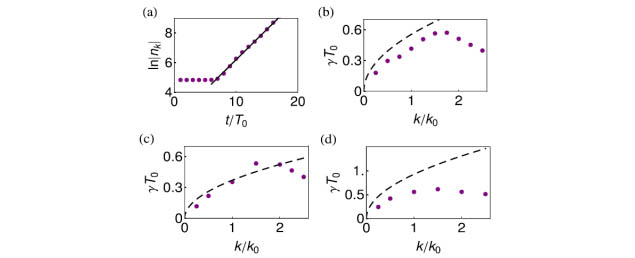
We are investigating strategies to reduce the degradation of the energy spectrum composite in the multispecies plasma target. For example in a hydrocarbon foil, the hydrogen ions (protons) quickly separate from the carbon ions and are accelerated on a stable interface, while shielded from the instability at the carbon-laser boundary.
Relevant Publications:
• V. Khudik, S. A. Yi, C. Siemon, and G. Shvets, "The analytic model of a laser-accelerated plasma target and its stability", Phys. Plasmas 21, 013110 (2014).
• V. Khudik, S. A. Austin, C. Siemon, and G. Shvets, "Monoenergetic acceleration of a target foil by circularly polarized laser pulse in RPA regime without thermal heating", AIP Conf. Proc. 1507, 803 (2012).
• T. P. Yu, A. Pukhov, G. Shvets, M. Chen, T. H. Ratliff, S. A. Yi, and V. Khudik, "Simulations of stable compact proton beam acceleration from a two-ion-species ultrathin foil," Phys. Plasmas 18, 043110 (2011).
• T. P. Yu, A. Pukhov, G. Shvets, and M. Chen, "Stable Laser-Driven Proton Beam Acceleration from a Two-Ion-Species Ultrathin Foil," Phys. Rev. Lett. 105, 065002 (2010).